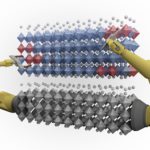
Precision Synthesis of Quantum and Energy Materials
A grand challenge for materials science is to design materials with tailored functionality. The controlled synthesis of a wide variety of oxide crystals via epitaxial growth is a powerful way to design thin films and heterostructures with atomic level precision, allowing us to produce materials with remarkable physical properties and functionalities. Spurred by recent advances in the synthesis of such artificial materials at the atomic scale, the physics of oxide heterostructures containing atomically smooth layers of such correlated electron materials with abrupt interfaces is a rapidly growing area. Thus, we have established a growth technique to control complex oxides at the level of unit cell thickness by pulsed laser epitaxy. The atomic-scale growth control enables to assemble the building blocks to a functional system in a programmable manner, yielding many intriguing physical properties that cannot be found in bulk counterparts. Functional complex-oxides display a wide spectrum of physical properties, including dielectricity, ferroelectricity, piezoelectricity, ferromagnetism, (semi)conducting behavior, and superconductivity. This class of functional materials, especially in forms of thin films and heterostructures, is strategically and industrially important and offers a wide range of opportunities for electronic, magnetic, piezoelectric, and thermoelectric applications, such as sensors, actuators, quantum computing, information storage, and energy storage and utilization. Specifically, our objectives are (1) to create functionalities in complex oxides by epitaxial stabilization, (2) to control order parameters, such as spin, electron, charge, and lattice, in interfacial materials to achieve novel functionalities, and (3) to understand topology and related quantum phenomena for advancing quantum materials for quantum information science. This is conducted by two epitaxial growth techniques: pulsed laser epitaxy of complex oxides and molecular beam epitaxy of chalcogenites and other metal-based topological materials.
References:
- C. Sohn, X. Gao, R. K. Vasudevan, S. M. Neumayer, N. Balke, J. M. Ok, D. Lee, E. Skoropata, H. Y. Jeong, Y.-M. Kim, and H. N. Lee, Strain-driven autonomous control of cation distribution for artificial ferroelectrics, Sci. Adv. 7, eabd7394 (2021).
- J. M. Ok, M. Brahlek, W. S. Choi, K. M. Roccapriore, M. F. Chisholm, S. Kim, C. Sohn, E. Skoropata, S. Yoon, J. S. Kim, and H. N. Lee, Pulsed-laser epitaxy of metallic delafossite PdCrO2 films, APL Mater. 8, 051104 (2020).
- D. Lee, X. Gao, L. Sun, Y. Jee, J. Poplawsky, T. O. Farmer, L. Fan, E.-J. Guo, Q. Lu, W. T. Heller, Y. Choi, D. Haskel, M. R. Fitzsimmons, M. F. Chisholm, K. Huang, B. Yildiz, and H. N. Lee, Colossal oxygen vacancy formation at a fluorite-bixbyite interface Nature Commun. 11, 1371 (2020).
- M. Brahlek, G. Rimal, J. M. Ok, D. Mukherjee, A. Mazza, Q. Lu, H. N. Lee, T. Z. Ward, R. R. Unocic. G. Eres, S. Oh, Growth of metallic delafossite PdCoO2 by molecular beam epitaxy, Phys. Rev. Mater. 3, 093401 (2019).
- W. S. Choi, S. Lee, V. Cooper, and H. N. Lee, Fractionally delta-doped oxide superlattices for higher carrier mobilities, Nano Lett. 19, 4590 (2012).
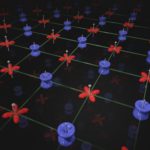
Emerging Quantum Phenomena and Physics of Symmetry, Correlation, and Topology
We study to understand the role of symmetry, strong electron correlation and topology on exotic physical phenomena to search for innovative quantum materials. The current focus is on controlling and utilizing symmetry, correlation, and topology to find many exotic quantum phenomena, such as skyrmions and quantum anomalous Hall effect. Correlated topological materials are also of interest based on recent investment on spin- and laser-ARPES as well as MBE.
References:
- J. M. Ok, N. Mohanta, J. Zhang, S. Yoon, S. Okamoto, E. S. Choi, H. Zhou, M. Briggeman, P. Irvin, A. R. Lupini, Y.-Y. Pai, E. Skoropata, C. Sohn, H. Li, H. Miao, R. G. Moore, B. Lawrie, W. S. Choi, G. Eres, J. Levy, and H. N. Lee, Correlated oxide Dirac semimetal in the extreme quantum limit, Sci. Adv. 7, eabf9631 (2021).
- H. Li, T. T. Zhang, T. Yilmaz, Y.-Y. Pai, C. Marvinney, A. Said, Q. W. Yin, C. S. Gong, Z. J. Tu, E. Vescovo, C. S. Nelson, R. G. Moore, S. Murakami, H. C. Lei, H. N. Lee, B. Lawrie, and H. Miao, Observation of unconventional charge density wave without acoustic phonon anomaly in kagome superconductors AV3Sb5 (A=Rb, Cs), Phys. Rev. X 11, 031050 (2021).
- C. Sohn, E. Skoropata, Y. Choi, X. Gao, A. Rastogi, A. Huon, M. A. McGuire, L. Nuckols, Y. Zhang, J. Freeland, D. Haskel, and H. N. Lee, Room-temperature ferromagnetic insulating state in highly cation-ordered double perovskite Sr2Fe1+xRe1-xO6 films, Adv. Mater. 31, 1805389 (2019).
- Z. Liao, M. Brahlek, J. M. Ok, L. Nuckols, Y. Sharma, Q. Lu, Y. Zhang, and H. N. Lee, Pulsed-laser epitaxy of topological insulator Bi2Te3 thin films, APL Mater. 7, 041101 (2019).
- Z. Liao, E. Skoropata, J. W. Freeland, E.-J. Guo, R. Desautels, X. Gao, C. Sohn, A. Rastogi, T. Z. Ward, T. Zhou, T. Charlton, M. R. Fitzsimmons, and H. N. Lee, Large orbital polarization in nickelate-cuprate heterostructures by dimensional control of oxygen coordination, Nature Commun. 10, 589 (2019).
- Y. Sharma, J. Balachandran, C. Sohn, J. T. Krogel, P. Ganesh, L. Collins, Q. Li, N. Balke, S. Kalinin, O. Heinonen, and H. N. Lee, Nanoscale control of metal-insulator transition in epitaxial vanadium dioxides, ACS Nano 12, 7159 (2018).
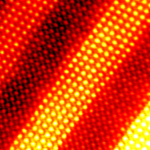
Physics of Oxide Interfaces and Hybrid Materials
References:
- E. Skoropata, J. Nichols, J. M. Ok, R. V. Chopdekar, E. S. Choi, A. Rastogi, C. Sohn, X. Gao, S. Yoon, T. Farmer, R. D. Desautels, Y. Choi, D. Haskel, J. W. Freeland, S. Okamoto, M. Brahlek, and H. N. Lee, Interfacial tuning of chiral magnetic interactions for large topological Hall effects in LaMnO3/SrIrO3 heterostructures, Science Adv. 6, eaaz3902 (2020).
- E. Guo, R. Desautels, D. Lee, M. A. Roldan, Z. Liao, T. Charlton, H. Ambaye, J. Molaison, R. Boehler, D. Keavney, A. Herklotz, T. Z. Ward, H. N. Lee, and M. R. Fitzsimmons, Exploiting symmetry mismatch to control magnetism in a ferroelastic heterostructure Phys. Rev. Lett. 122, 187202 (2019).
- E.-J. Guo, R. D. Desautels, D. Keavney, M. A. Roldan, B. J. Kirby, D. Lee, Z. Liao, T. Charlton, A. Herklotz, T. Z. Ward, M. R. Fitzsimmons, and H. N. Lee, Nanoscale ferroelastic twins formed in strained LaCoO3 films, Sci. Adv. 5, eaav5050 (2019).
- J. S. Kim, S. S. A. Seo, M. F. Chisholm, R. K. Kremer, H.-U. Habermeier, B. Keimer, and H. N. Lee, Nonlinear Hall effect and multichannel conduction in LaTiO3/SrTiO3 superlattices, Phys. Rev. B 201407(R) (2010).
- H. N. Lee, H. M. Christen, M. F. Chisholm, C. M. Rouleau, and D. H. Lowndes, Strong polarization enhancement in asymmetric three-component ferroelectric superlattices, Nature, 433, 395 (2005).
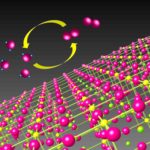
Energy Materials – Functional Defects, Ionic Oxides, and Oxygen Sponges
- E.-J Guo, Y. Liu, C. Sohn, R. D. Desautels, A. Herklotz, Z, Liao, J. Nichols, J. W. Freeland, M. R. Fitzsimmons, and H. N. Lee, Oxygen diode formed in nickelate heterostructures by chemical potential mismatch, Adv. Mater. 1705904 (2018).
- J. Petrie et al., Enhancing perovskite electrocatalysis through strain tuning of the oxygen deficiency, J. Am. Chem. Soc. 138, 7252 (2016).
- J. Petrie et al., Enhanced bifunctional oxygen catalysis in strained LaNiO3 perovskites, J. Am. Chem. Soc. 138, 2488 (2016).
- H. Jeen et al., Reversible redox reactions in an epitaxially stabilized SrCoOx oxygen sponge, Nature Materials 12, 1057 (2013).
- W. S. Choi, et al., Reversal of the lattice structure in SrCoOx epitaxial thin films studied by real-time optical spectroscopy and first-principles calculations, Phys. Rev. Lett. 111, 097401 (2013).
- H. Jeen et al., Topotactic phase transformation of the brownmillerite SrCoO2.5 to the perovskite SrCoO3-δ, Adv. Mater. 25, 3651 (2013).
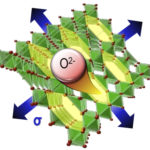
Strain Engineering of Oxide Thin Films
- T. Meyer, R. Jacobs, D. Lee, L. Jiang, J. W. Freeland, C. Sohn, T. Egami, D. Morgan, and H. N. Lee, Strain control of oxygen kinetics in the Ruddlesden–Popper oxide La1.85Sr0.15CuO4, Nature Commun. 9, 92 (2018).
- [Featured Review] A. Herklotz, D. Lee, E.-J. Guo, T. L. Meyer, J. R. Petrie, and H. N. Lee, Strain coupling of oxygen nonstoichiometry in perovskite thin films, J. Phys.: Condens. Matter 29, 493001 (2017).
- J. R. Petrie, C. Mitra, H. Jeen, W. S. Choi, T. Meyer, F. A. Reboredo, J. W. Freeland, G. Eres, and H. N. Lee, Strain control of oxygen vacancies in epitaxial strontium cobaltite films, Adv. Funct. Mater. 26, 1564 (2016).
- K. A. Stoerzinger, W. S. Choi, H. Jeen, H. N. Lee, and Y. Shao-Horn, Role of Strain and conductivity in oxygen electrocatalysis on LaCoO3 thin films, J. Phys. Chem. Lett. 6, 487 (2015).
- W. S. Choi, J.-H. Kwon, H. Jeen, J. E. Hamann-Borrero, A. Radi, S. Macke, R. Sutarto, F. He, G. A. Sawatzky, V. Hinkov, M. Kim, and H. N. Lee, Strain-induced spin states in atomically ordered cobaltites, Nano Lett. 12, 4966 (2012).
- H. N. Lee, S. M. Nakhmanson, M. F. Chisholm, H. M. Christen, K. M. Rabe, and D. Vanderbilt, Suppressed dependence of polarization on epitaxial strain in highly polar ferroelectrics, Phys. Rev. Lett. 98, 217602 (2007).
- H. N. Lee, H. M. Christen, M. F. Chisholm, C. M. Rouleau, and D. H. Lowndes, Strong polarization enhancement in asymmetric three-component ferroelectric superlattices, Nature, 433, 395 (2005).